Autonomous Vehicles: Uncertainties and Energy Implications
Release Date: 5/30/18
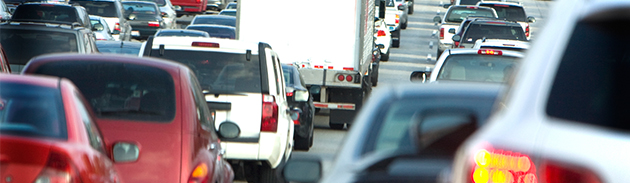
The potential effect autonomous vehicles may have on the future of personal mobility and freight movement has received considerable attention in recent years. This attention has focused not only on discussion of real and perceived costs of travel, shared-use mobility [1], travel patterns, vehicle design, and vehicle ownership, but also on how autonomous vehicles may affect energy markets. [2] This article uses modeling results from scenarios related to the Annual Energy Outlook 2018 (AEO2018) to help quantify the potential effects on energy of widespread adoption of autonomous vehicles. The widespread adoption of autonomous vehicles is uncertain, and these scenarios assume that purchasing an autonomous vehicle is favorable for both households and fleet operators.
The Autonomous Battery Electric Vehicle case assumes more widespread use of light-duty autonomous vehicles than the Reference case and that these vehicles are increasingly battery electric. The Autonomous Hybrid Electric Vehicle case also assumes more widespread use of light-duty autonomous vehicles than the Reference case and that these vehicles are increasingly hybrid electric. In both cases, households and shared-use mobility providers purchase autonomous vehicles, mass transit use is affected, and fleet operated long-haul trucking begins using automation technology.
Transportation energy demand in 2050 is higher in both scenarios compared with the Reference case but still remains lower than 2017 transportation energy demand. Energy use from higher light-duty vehicle miles traveled are partially offset by greater fuel efficiency from rising sales of more energy-efficient battery electric and hybrid electric vehicles. The changing sales mix by vehicle powertrain type affects transportation fuels, and motor gasoline and electricity use vary among the Reference and scenario side cases. In addition, more widespread use of autonomous vehicle technology changes the energy consumption by mass transit modes and, more modestly, by trucking.
These scenarios are assumption driven and do not cover the full range of uncertainties surrounding the effects that autonomous vehicles could have on energy.
Background
In this article, definitions of varying levels of vehicle automation and the potential benefits it provides and obstacles it faces are addressed. The potential effects on transportation energy markets of autonomous vehicles are also briefly discussed.
Definitions of vehicle automation
The U.S. Department of Transportation defines vehicle automation as vehicles that have at least some aspects of safety-critical control functions (such as steering, throttle, or braking) that occur without driver input. [3] Vehicle automation is divided into six levels, which denote increasing autonomous capability (Figure 1). Autonomous vehicles are a subset of automation at Levels 4 and 5. Level 4 automation is free of human driving in defined areas and under certain conditions, and a human driver must take control outside of those conditions. Level 5 is free of a human driver in all operating circumstances. Autonomous vehicle self-driving capability is possible with limited or no communication with other vehicles, infrastructure, or a cloud network. The complete mix of vehicles with varying communication and automated technologies is referred to as connected and autonomous vehicles (CAV). Unless otherwise noted, the term autonomous vehicles in this article refers to those with Level 4 or 5 capabilities and does not distinguish between autonomous and connected autonomous vehicles.
Automakers, suppliers, and technology companies are currently researching, developing, and testing autonomous technologies and vehicles. Trials are already underway, and the United States is playing a leading role in early testing. At the end of 2017, Level 4 automation began testing without a human in the driver seat on public roads. [4] Companies are looking to expand the use of autonomous vehicles into shared-use mobility applications on limited, well-mapped routes later in 2018. Timelines for sale to individual consumers range from the mid-2020s to mid-2030s. However, early testing resulted in accidents, which has resulted in uncertainty about expanded use on public roads.
Source: U.S. Department of Transportation, Automated Driving Systems 2.0, A Vision for Safety
Potential benefits
Many studies point to the potential societal and individual benefits that underlie the interest in adopting autonomous vehicles. These benefits will likely increase as adoption rises, highlighting the importance of consumer and regulatory acceptance, and require increased connectivity to achieve the highest potential. Further, it could take several decades for technologies introduced in newer vehicles to gradually become a large share of the entire vehicle stock. [5]
Road safety may be one of the primary benefits of autonomous vehicle use. In 2016, motor vehicle-related crashes killed more than 37,000 people and injured millions more. According to the U.S. Department of Transportation, 94% of all serious crashes are a result of dangerous choices or errors people make behind the wheel. [6] Autonomous vehicles and lower-level automated technology could reduce the number of traffic accidents, fatalities, and injuries by lowering or removing human error.
Autonomous vehicles may also provide increased mobility for the elderly, youth, and people with mobility-inhibiting disabilities. According to estimates from the U.S. Census Bureau, about 20% of the U.S. population is either between the ages of 10 and 17 or 70 years and older. [7] In addition, about 10% of the U.S. population between the ages of 18 and 64 claim some form of disability, although the number of those disabilities that inhibit driving is unknown. Expanding personal vehicle mobility options to include people who cannot obtain a driver’s license or who face physical challenges with driving could increase their quality of life.
Autonomous vehicles, especially CAVs, could improve traffic flow and reduce accidents, both of which are sources of vehicle congestion. In 2014, congestion increased urban Americans’ travel time by 6.9 billion hours, which resulted in purchasing 3.1 billion gallons more fuel at a cost of $160 billion. [8] Autonomous vehicle use as part of an intelligent transportation system could provide vehicle occupants and freight with a reliable, optimized, less congested pathway from origin to destination.
The average American driver spends 294 hours behind the wheel per year. [9] In aggregate, the nation’s 222 million drivers spend about 65 billion hours driving their vehicles. [10] Autonomous vehicles could not only reduce traffic congestion and time spent on the road, but also provide time for drivers to engage in other activities.
Potential obstacles
Although the widespread use of autonomous vehicles has significant benefits, many studies also highlight uncertainties and obstacles.
Most people do not like the idea of giving up control of their vehicles. According to a study by the American Automobile Association (AAA), 75% of members surveyed reported feeling afraid to ride in a self-driving vehicle. [11] However, this same study also found that many consumers are interested in semi-autonomous Level 1 or Level 2 vehicles and claimed that gradual experience with this semi-autonomous vehicle technology could ease fear of self-driving autonomous vehicles. Similarly, a 2018 Gallup poll found that 54% of the U.S. public are unlikely to use self-driving cars, and 59% would be uncomfortable riding in a self-driving car, although younger adults and people with higher levels of education expressed more willingness to use or ride in a self-driving car. [12] Of note, Gallup highlighted how people underestimate their potential to accept new technology. For example, 23% of U.S. adults surveyed in 2000 expressed that they would never get a cellphone. Other studies highlight the generational differences in acceptance of autonomous vehicle technology. [13]
The additional costs associated with autonomous vehicles could affect commercial and consumer adoption. Autonomous vehicles require sophisticated on-board hardware and software and may require information technology for connected autonomous vehicles. No definitive data exist on how much the requisite sensory or connectivity technologies cost today, nor how far these costs could fall in the future. In addition, there is no definitive set of technologies for autonomous vehicles of the future. Level 1 and Level 2 systems are already broadly available, costing between $1,500 and $3,000 per vehicle. Level 4 autonomous test vehicles are not affordable for the average consumer. A 2012 study by J.D. Power and Associates found that the percentage of drivers who expressed interest in purchasing a fully autonomous vehicle fell by half once told of added costs. [14] Critical technologies are developing quickly. As a result, costs are changing and will likely fall with higher-volume production because of economies of scale and other breakthroughs.
Cybersecurity is also an important consideration as it relates to both trust and acceptance of CAV technology and safety. Like all other connected communications networks, concerns remain that hackers could steal personal information and spy on people or that malicious control of a vehicle could cause personal harm or disrupt traffic flow. [15]
A legal framework will be necessary to address future liability issues related to autonomous vehicles. At present, it is unknown how the assignment of fault in a vehicle accident could shift when the vehicle is not controlled by an individual driver. Fault and liability for damages could belong to automakers, autonomous vehicle equipment manufacturers, software providers, owners of the autonomous vehicle, or others such as drivers of non-autonomous vehicles. Courts will resolve these complex issues for users and insurance providers, but legal delays and outcomes could affect the deployment and availability timelines.
Autonomous vehicles would require infrastructure needs, such as well-maintained signs and road markings. Further, CAVs could benefit from interaction with infrastructure. There may be cost barriers for governments at all levels to provide enabling infrastructure. In addition, if autonomous vehicles become more widespread in the future, infrastructure requirements could be different than today, which has unknown cost implications. These unknowns include the uncertain effect that increased use of autonomous vehicles will have on public mass transit systems.
The uncertainties in adoption and availability involve important policy decisions as well. At this early stage, few regulations govern or restrict the use of autonomous vehicles. Citing the potential for safety, increased mobility, and economic benefits, lawmakers and regulatory agencies are allowing autonomous vehicle testing. As the use of autonomous vehicles becomes more widespread and important policy factors become clearer, this legal position could change. The course of such changes is unknown and could affect the pace of autonomous vehicle deployment.
Potential energy effects
On-road vehicles in the United States are significant users of energy, making the potential ramifications of autonomous vehicles on energy an important factor. In 2017, on-road vehicles in the United States, including light-duty passenger cars and trucks, buses, and commercial and freight trucks, consumed 22.1 quadrillion British thermal units (Btu), or 11.6 million barrels per day (b/d) oil equivalent, which accounted for 80% of all transportation energy use and 31% of all delivered end-use energy in the United States. Light-duty passenger cars and trucks (LDVs) used 15.3 quadrillion Btu, or 8.3 million b/d oil equivalent, which was 21% of total delivered end-use energy consumption in the United States.
Petroleum-based liquid fuels continue to dominate the fuels used in LDVs and in the transportation sector more broadly. Changes in energy demand as a result of increased use of autonomous light-duty vehicles could significantly affect petroleum product markets, particularly motor gasoline, which includes ethanol blended with gasoline. In 2017, 99% of the energy used by LDVs was motor gasoline, which accounted for 88% of all the motor gasoline used in the United States. Autonomous vehicle technology may also affect diesel, the second most-used on-road vehicle fuel, especially for heavy-duty freight trucks, which consumed 61% of total U.S. diesel in 2017.
The effect of autonomous vehicles on transportation energy consumption is highly uncertain. Recent literature on the potential effects on energy as a result of adopting autonomous vehicles shows that U.S. LDV energy use could decrease by around 60% or could increase by 200%. [16] For perspective, applying the two extremes to U.S. LDV energy consumption in 2017 (15.3 quadrillion Btu, or 8.3 million b/d oil equivalent) gives an estimated consumption between 6.1 quadrillion Btu, or 3.3 million b/d oil equivalent, and 45.9 quadrillion Btu, or 24.9 million b/d oil equivalent.
Travel demand and energy efficiency. The wide range of potential effects on energy is a direct result of the uncertain effect of autonomous vehicles on LDV travel demand and energy efficiency. [17] A significant decrease in LDV energy use is possible if major energy efficiency gains are realized without much change in vehicle miles traveled, and a large increase in LDV energy consumption is possible if much greater LDV miles traveled are accompanied by relatively few gains in energy efficiency.
Autonomous vehicles could affect vehicle travel demand and energy efficiency in many ways (Table 1). The ranges for each factor highlight the uncertainties inherent in these estimations. Some factors are specific to an on-road mode or whether the vehicle is driven in cities, highways, or rural roads. Several factors depend on or may require a relatively high number of other autonomous vehicles on the road. Finally, many of these factors are interrelated. For example, cheaper travel, either through improved energy efficiency or reduced perceived individual cost of travel, could increase congestion and waste time and fuel consumed, and eventually, travel demand may decrease through a rebound effect.
Determinant | Factor (with source) |
Range of effect less energy / more energy | Mode | Drive type | Penetration |
---|---|---|---|---|---|
Travel | less hunting for parking (2) | -5% to -11% | LDV | city | n/a |
Travel | ridesharing (2) | 0% to -12% | LDV | city | n/a |
Travel | Mobility as a Service (1) | 0% to -20% | LDV | any | any |
Travel | empty miles (2) | 0% to +11% | LDV | city | n/a |
Travel | underserved population (1) | +2% to +10% | LDV | any | any |
Travel | underserved population (2) | +2% to +40% | LDV | any | n/a |
Travel | mode switch (walk/transit/regional air) (2) | +7% | LDV | any | n/a |
Travel | reduced perceived cost of driving (1) | +6% to +60% | LDV + HDV | any | any |
Travel | ease of travel (traffic flow/crash avoidance/reduced cost of driving) (2) | +20% to +160% | LDV | any | n/a |
Efficiency | eco-driving (1) | 0% to -20% | LDV + HDV | city | any |
Efficiency | congestion mitigation (1) | -2% to -4% | LDV + HDV | city | medium-high |
Efficiency | collision avoidance (2) | 0% to -2% | LDV | any | n/a |
Efficiency | drive profile + traffic flow smoothing (2) | -5% to -23% | LDV | any | n/a |
Efficiency | intersection-vehicle communication (2) | -2% to -6% | LDV | any | n/a |
Efficiency | platooning (1) | -3% to -25% | LDV | highway | any |
Efficiency | platooning (2) | -13% to -25% | LDV | highway | n/a |
Efficiency | de-emphasize performance (1) | -5% to -23% | LDV | any | any |
Efficiency | smaller vehicles (crash avoidance) (1) | -6% to -23% | LDV | any | high |
Efficiency | platooning (1) | -10% to -25% | HDV | highway | any |
Efficiency | “right-sizing” of vehicles (1) | -21% to -45% | LDV | any | high |
Efficiency | vehicle/powertrain resizing (2) | 0% to -50% | LDV | any | n/a |
Efficiency | faster travel (2) | 0% to +40% | LDV | highway | n/a |
Efficiency | faster travel (1) | +7% to +22% | LDV | any | any |
Efficiency | increased feature content (1) | 0% to +11% | LDV | highway | medium-high |
Notes: Similar factors may be listed differently for each study. Source: (1) Help or Hindrance? The Travel, Energy, and Carbon Impacts of Highly Automated Vehicles, Zia Wadud et al, Transportation Research Part A 86 (2016) 1-18. U.S. Energy Information Administration, Office of Energy Analysis. (2) Estimated Bounds and Important Factors for Fuel Use and Consumer Costs of Connected and Automated Vehicles, Tom Stephens et al, National Renewable Energy Laboratory Technical Report NREL/TP-5400-67216, November 2016. |
Alternative fuels and energy-efficient powertrains. Autonomous vehicles could promote the sale of more energy-efficient or alternatively fueled vehicles through a faster payback of the more expensive purchase price. Shared-use mobility providers, such as taxis or transportation network companies, offer the greatest potential for a faster payback. Although private household vehicles are driven about 12,000 miles per year on average, shared-use mobility providers typically drive significantly more. Other cost considerations are also important, such as differences in maintenance, repair, fueling infrastructure, faster vehicle replacement because of increased usage, and the cost of the autonomous vehicle technology.
Autonomous vehicles could promote the use of alternative fuels through refueling without the rider, ensuring that riders avoid having to search for non-motor gasoline refueling infrastructure. Autonomous vehicles could reduce range anxiety related to plug-in electric vehicles by ensuring that consumers always have an electric vehicle available with sufficiently remaining charge. However, not much analysis has been done on the obstacles to alternatively fueled autonomous vehicles. A major obstacle is sufficient refueling infrastructure, for example, the amount of time and space required to charge heavily driven electric vehicles and the additional costs of the larger plug-in electric vehicle batteries required for the increased energy draw from autonomous technology and additional onboard consumer infotainment.
Commercial trucks. Commercial light- and heavy-duty trucks accounted for 23% of transportation energy demand in 2017, or 6.5 quadrillion Btu (3.2 million b/d oil equivalent). Further, commercial trucks are projected to account for 27% of transportation energy consumption by 2050 in the AEO2018 Reference case. According to the U.S. Department of Energy, widespread adoption of platooning operations in heavy-duty vehicles (HDVs), where groups of vehicles travel in a tight formation to reduce aerodynamic drag, could potentially reduce truck energy use by 4% compared to today’s levels. [18] The addition of autonomous technology to commercial trucks could also include alternative fuels to diesel, which raises questions similar to those for LDVs. The greatest uncertainty lies in how autonomous vehicles could affect the cost to ship goods, which may alter freight and parcel markets and overall truck travel demand. Automated trucks could operate continuously, potentially reducing downtime and changing logistics for total truck demand and changing shipping costs. These effects would be most pronounced in diesel, with 81% of 2017 U.S. distillate fuel oil consumed in transportation.
Mass transit. Little research has been done to examine the potential effects on energy of autonomous vehicles on mass transit systems, including passenger rail and bus. Issues to consider include whether adding autonomous technologies to mass transit and automated vehicles would take away from or complement mass transit. For example, autonomous LDVs could increase the use of mass transit if they are offered via consumer programs that integrate various modes of transportation though unified trip management. Conversely, autonomous LDVs could become a competitor to conventional mass transit systems. Moving people away from highly energy-efficient mass transit modes and toward individual autonomous light-duty vehicles could have significant energy demand implications.
Description of scenarios
The AEO2018 includes two cases examining potential energy implications from more widespread use of autonomous vehicles compared with the Reference case. As discussed, there is uncertainty surrounding extensive deployment of autonomous vehicles.
For widespread adoption of autonomous vehicles to occur, they must offer vehicle purchasers a positive value proposition, meaning the benefits gained from having an autonomous vehicle are greater than related costs. Household consumers may see this balance as a decision between the additional value of potential benefits such as safety, ease of travel, or time savings versus the incremental vehicle technology cost and concern about giving up control of driving. Further, future decisions will consider the benefits and costs of purchasing a less automated Level 1 through Level 3 vehicle compared with purchasing a Level 4 or Level 5 autonomous vehicle.
The decision to purchase an autonomous vehicle by fleet-operated shared-use mobility providers is more about the financial value of profits and expenses, but the decision also includes consideration of the benefits and obstacles facing autonomous vehicles.
The two cases assume that the value proposition is positive enough for both household consumers and fleet operators to purchase autonomous vehicles much more than in the Reference case. These cases are assumption-driven and do not cover the full range of uncertainty surrounding the energy effects of autonomous vehicles (Table 2). The different cases explore how greater personal mobility, enabled by autonomous vehicles, has energy implications that differ depending on if these vehicles are increasingly battery electric vehicles (BEVs) or hybrid-electric vehicles (HEVs), as well as interactions with several mass transit modes that differ from the Reference case. Both cases also include identical assumptions on operational efficiency and platoonable mileage associated with long-haul freight fleet trucks. [19]
Case name | Assumptions | Description |
---|---|---|
Reference | Autonomous vehicles enter fleet light-duty vehicles |
|
Autonomous vehicles are used more intensively |
|
|
Autonomous light-duty vehicle fuel type |
|
|
Autonomous light-duty vehicles affect mass transit modes |
|
|
Autonomous Battery Electric Vehicle | Autonomous vehicles enter household and fleet light-duty vehicles |
|
Autonomous vehicles are used more intensively |
|
|
Autonomous light-duty vehicle fuel type |
|
|
Autonomous light-duty vehicles affect mass transit modes |
|
|
Autonomous freight truck platooning by long-haul fleets |
|
|
Autonomous Hybrid Electric Vehicle | Autonomous vehicles enter household and fleet light-duty vehicles |
|
Autonomous vehicles are used more intensively |
|
|
Autonomous light-duty vehicle fuel type |
|
|
Autonomous light-duty vehicles affect mass transit modes |
|
|
Autonomous freight truck platooning by long-haul fleets |
|
|
Autonomous vehicle case descriptions
The autonomous vehicle cases have similar assumptions except for the autonomous light-duty vehicle fuel type used among them. The following descriptions highlight changes from Reference case assumptions for each scenario.
Autonomous Battery Electric Vehicle case
The AEO2018 Autonomous Battery Electric Vehicle case assumes more widespread use of autonomous vehicles than in the Reference case. In the Reference case, autonomous vehicles are used in shared-use mobility provided by fleet operators, such as transportation network companies. The Autonomous Battery Electric Vehicle case increases the number of autonomous vehicles used by fleets and also introduces them into households, allowing increased personal mobility through vehicle miles traveled. In this side case, an increasing share of new autonomous vehicle sales by 2050 are BEVs, while in the Reference case autonomous vehicles are conventional internal combustion gasoline engine vehicles. Mass transit modes are affected—transit rail use is reduced by the competition while commuter rail use increases because autonomous vehicles allow greater connection. Transit bus use declines at first because of competition for passengers, but it rebounds once autonomous transit buses become common. Large-fleet long-haul freight trucks start using platooning to improve fuel efficiency, reaching 50% of fleet long-haul freight-truck travel by 2050.
Autonomous Hybrid Electric Vehicle case
The AEO2018 Autonomous Hybrid Electric Vehicle case has the same assumptions as the AEO2018 Autonomous Battery Electric Vehicle case except fuel choice. An increasing share of new autonomous light-duty vehicle sales is gasoline hybrid-electric internal-combustion engine vehicles by 2050.
Results
Transportation energy demand is higher in both the Autonomous Battery Electric Vehicle case and the Autonomous Hybrid Electric Vehicle case compared with the Reference case (Figure 2). By 2050, transportation energy consumption in the Autonomous Battery Electric Vehicle case is 26.3 quadrillion Btu (13.4 million b/d oil equivalent), 3% higher than in the Reference case. It reaches 26.6 quadrillion Btu (13.6 million b/d oil equivalent) in the Autonomous Hybrid Electric Vehicle case, 4% higher than in the Reference case.
Despite these relative increases compared with the Reference case, energy demand in both side cases in 2050 is still lower than 2017 transportation energy consumption. Transportation energy consumption in the Autonomous Battery Electric Vehicle case is 5% lower in 2050 than in 2017, the Autonomous Hybrid Electric Vehicle case is 4% lower, and the Reference case is 8% lower.
Transportation fuels
Although both cases show higher energy consumption across the projection compared with the Reference case, there are important differences in the fuels used in transportation (Figure 3). Given the higher penetration of BEVs in the Autonomous Battery Electric Vehicle case, motor gasoline demand in transportation is nearly identical in the Reference case in 2050, at 11.4 quadrillion Btu, and demand for electricity is significantly higher, reaching 1.3 quadrillion Btu. In the Autonomous Hybrid Electric Vehicle case, motor gasoline consumption is higher than in the Reference case in 2050, reaching 12.5 quadrillion Btu. Diesel consumption is nearly identical to the Reference case in both cases because of limited assumptions about platooning in commercial vehicles.
Light-duty vehicles
Light-duty vehicle energy consumption is higher in both side cases compared with the Reference case (Figure 4). In 2050, LDV consumption in the Autonomous Battery Electric Vehicle case is 11.5 quadrillion Btu (6.2 million b/d oil equivalent), 7% higher than in the Reference case, and the Autonomous Hybrid Electric Vehicle case reaches 11.9 quadrillion Btu (6.4 million b/d oil equivalent), 10% higher than in the Reference case. Yet, energy consumption levels in 2050 remain far lower than 2017 levels: 25% lower in the Autonomous Battery Electric Vehicle case and 23% lower in the Autonomous Hybrid Electric Vehicle case, compared with 29% lower in the Reference case.
LDV energy demand is higher because of the increase in light-duty vehicle miles traveled (Figure 5). In both cases, vehicle miles traveled are 14% higher in 2050 than in the Reference case, reaching almost 3.8 trillion miles, compared with 3.3 trillion miles in the Reference case. Importantly, vehicle miles traveled in both cases are 35% higher in 2050 than in 2017, versus 18% higher in the Reference case.
Higher light-duty vehicle miles traveled occur with a relatively smaller increase in energy consumption, because this greater mobility is offset by higher fuel efficiency. This increased fuel efficiency comes from the growing use of much more fuel-efficient autonomous BEVs and HEVs (Figure 6). In the Autonomous Battery Electric Vehicle case, BEVs account for 31% of new LDV sales in 2050, compared with 12% in the Reference case. In the Autonomous Hybrid Electric Vehicle case, HEVs reach 27% of new LDV sales in 2050, compared with 5% in the Reference case.
New conventional gasoline internal combustion vehicle fuel economy is about 48 miles per gallon for passenger cars and about 39 miles per gallon for light-duty trucks in 2050. These levels compare with fuel economy of about 110 miles per gallon equivalent for battery electric passenger cars, about 90 miles per gallon equivalent for battery electric light-duty trucks, about 70 miles per gallon for hybrid electric passenger cars, and about 57 miles per gallon for hybrid electric light-duty trucks.
Increasing sales of higher fuel economy light-duty autonomous vehicles raise total new LDV fuel economy (Figure 7). New light-duty compliance fuel economy grows from 33 miles per gallon in 2017 to 49 miles per gallon in 2050 in the Reference case, to 53 miles per gallon in the Autonomous Battery Electric Vehicle case, and to 51 miles per gallon in the Autonomous Hybrid Electric Vehicle case.
Mass transit modes
Widespread use of autonomous vehicles affects energy consumption by mass transit modes in the two side cases by enabling greater usage of commuter rail through autonomous vehicle connection and reducing the use of transit rail and transit bus through autonomous vehicle competition. Transit bus usage decreases until the mid-2030s before increasing, when autonomous transit buses become prevalent (Figure 8). Energy consumption by commuter rail is almost 50% higher in 2050 in both side cases compared with the Reference case. Transit rail sees a 35% reduction in energy demand in 2050 compared with the Reference case. Transit bus energy demand declines sharply before rising nearly 50% higher than in the Reference case in 2050. Mass transit energy consumption is a relatively small share of transportation energy consumption, and these changes have a minor effect on overall transportation energy demand.
Freight trucks
Only modest changes are included in these scenarios regarding the effect of autonomous vehicle technology on freight trucks. When platooning technologies are included, the assumption is an operational fuel efficiency improvement of 7%, but this assumption is included only for fleet-operated class 7 and class 8 trucks, a relatively small share of the total heavy-duty freight truck market and travel. Further, these improvements are offset by higher freight travel demand because of shipments related to changes in petroleum product demand, which results in energy consumption that is nearly identical to the Reference case.
Endnotes
-
Shared-use mobility includes all transportation services that are shared among users, such as public transit, ridesourcing, transportation network companies, and taxis. U.S. Department of Transportation, Federal Transit Administration, Shared Mobility Definitions. Accessed April 11, 2018.
-
U.S. Energy Information Administration, Study of the Potential Energy Consumption Impacts of Connected and Automated Vehicles, March 30, 2017, accessed April 4, 2018.
-
U.S. Department of Transportation, Automated Driving Systems 2.0, A Vision for Safety, accessed April 6, 2018.
-
“Humans take back seat as Waymo’s Driverless cars pass Phoenix milestone” Richard Waters, Financial Times, November 8, 2017.
-
Predicted availability of safety features on registered vehicles—an update. Highway Loss Institute, Bulletin Vol. 31, No. 15, 2014.
-
U.S. Department of Transportation, National Highway Traffic Safety Administration, Automated Vehicles for Safety, accessed December 15, 2017.
-
U.S. Census Bureau, 2015 ACS 5-year Estimates, accessed December 15, 2017.
-
Texas A&M Transportation Institute, Texas A&M University System, INRIX, Inc., 2015 Urban Mobility Scorecard, August 2015, accessed December 18, 2017.
-
American Automobile Association (AAA), American Driving Survey 2014-2015
-
U.S. Department of Transportation, Federal Highway Administration, Highway Statistics 2016, accessed December 18, 2017.
-
“Three-Quarters of Americans ‘Afraid’ to Ride in a Self-Driving Vehicle”. Erin Stepp, AAA NewsRoom, March 1, 2016.
-
“Americans Hit the Brakes on Self-Driving Cars”. RJ Reinhart, February 21, 2018.
-
Autonomous Vehicles, Trust, and Driving Alternatives: A Survey of Consumer Preferences Hilary Abraham et al, Massachusetts Institute for Technology, AgeLab, May 2016.
-
U.S. Energy Information Administration, Study of the Potential Energy Consumption Impacts of Connected and Automated Vehicles, March 30, 2017, accessed April 4, 2018.
-
“The Jeep Hackers are Back to Prove Car Hacking Can Get Much Worse”. Andy Greenberg, Wired, January 2018; “Can a Connected Car Ever Be Safe from hacking?” Jared Gall, Car and Driver, October 2017.
-
Estimated Bounds and Important Factors for Fuel Use and Consumer Costs of Connected and Automated Vehicles, Tom Stephens et al, National Renewable Energy Laboratory Technical Report NREL/TP-5400-67216, November 2016.
-
U.S. Energy Information Administration, Study of the Potential Energy Consumption Impacts of Connected and Automated Vehicles, March 30, 2017, accessed April 4, 2018
-
U.S. Department of Energy, National Renewable Energy Laboratory, Potentials for Platooning in U.S. Highway Freight Transport, January 2017, accessed March 4, 2018.
-
U.S. Department of Energy, National Renewable Energy Laboratory, Potentials for Platooning in U.S. Highway Freight Transport, January 2017, accessed March 4, 2018.